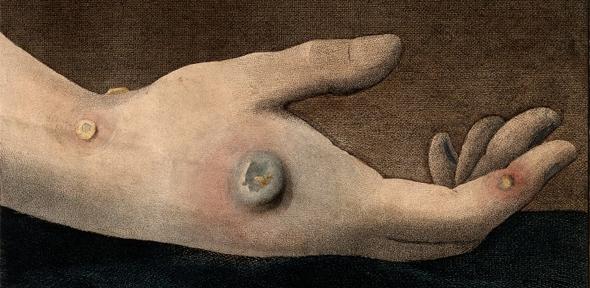
This is a tale of subterfuge and double agents, of armed struggle against an invading force and of defensive weapons being turned against their makers. But these events are happening much closer to home than you could ever imagine—these are battles fought every day within our very own bodies.
The protagonists in this struggle are viruses, which seek to exploit the resource-rich environment of our cells, and every single cell in our body, whose very survival depends on their ability to repel these viral invaders.
Viruses are masters of subterfuge, blagging their way into cells and once inside hijacking the cell’s resources in a relentless quest to generate more copies of themselves. Cells possess innate defences against viral colonisation: they can raise the alarm, warning nearby cells when they have succumbed to infection, and can sacrifice themselves, depriving the virus of a host. But recent work from the Department of Pathology in Cambridge has revealed that poxviruses have acquired genes that once formed part of the host cell’s immune defences and have turned them to serve the virus’s own ends, acting as double-agents to deceive our immune system.
When it comes to the subtle art of immune evasion poxviruses are undoubtedly masters. The most notorious poxvirus is variola virus, which caused smallpox until its successful eradication in 1979 following a heroic immunisation campaign by the World Health Organisation. The DNA genomes of poxviruses contain roughly 200 genes. While this is many more than Ebola virus, which gets by with just seven genes in total, it is dwarfed by the 20,000 or so genes that make up you and me.The poxvirus genome encodes all the components necessary for poxviruses to invade cells, make abundant copies of themselves, and ensure these copies escape the infected cells so they can go off and colonise further cells. However, half of these 200 genes exist for the sole purpose of thwarting the body’s attempts to prevent infection or to limit the damage caused once infection is established.
Perhaps the most dramatic response of our cells to viral infection is to self-destruct, depriving the virus of a host and thus stopping the infection from spreading. This selfless act is called “apoptosis”. The triggering of apoptosis is regulated in our bodies by a finely tuned system of pro-death and pro-survival molecules. Unsurprisingly, viruses want to defuse the cell’s apoptosis bomb and make sure host cells survive long enough for the virus to replicate. When my colleagues determined the three-dimensional structure of the poxvirus protein called N1 they saw that it looked almost identical to the cellular pro-survival molecules that regulate apoptosis. Our studies showed that N1 is indeed a viral ‘apoptosis bomb disposal expert’ – it functions as a pro-survival gene, ensuring that cells refrain from self-destruction even when they know that they are infected.
But inhibiting apoptosis is not the only means by which poxviruses deter the host’s attempts to limit viral infection. When cells realise they have become infected by a virus they produce messenger molecules to warn nearby cells of the imminent danger. They do this by turning on transcription factors—regulators of gene activity. One transcription factor, NF-κB, is the Big Red Button of cellular signalling: when this is switched on the full armada of the body’s immune defences is recruited to the site of infection. It is roughly equivalent to the cell calling in air support.
Recently we solved the three-dimensional structure of a poxvirus protein called A49 that jams the NF-κB signal. Amazingly, we found that A49 also looks very similar to the cellular pro-survival molecules that regulate apoptosis, despite having a completely different function in cells. Furthermore, we also found that the protein we’d previously studied, N1, is able to jam the NF-κB signal in addition to its ability to block apoptosis.
Our findings with N1, A49 and other poxvirus proteins led us to wonder: is the similarity of these proteins to cellular pro-survival molecules more than just a coincidence? We know that the structures of proteins are conserved when those proteins descend from a common ancestor gene; it’s like the facial similarities you’d expect if looking through a family photo album.
To test whether the poxvirus proteins share a common ancestor, we systematically compared them with all the cellular pro-survival molecules that regulate apoptosis. We found that all poxvirus proteins do indeed lie on a single branch of this evolutionary tree, distinct from the branches containing host-cell proteins. This suggests that an ancestral poxvirus first acquired from its host a gene that had pro-survival activity against apoptosis, and that over the course of the evolutionary struggle between poxviruses and their unwelcoming hosts the virus has duplicated this gene and adapted it to have additional, useful functions.
Our work emphasises how evolutionary pressure can very finely tune a virus to the immune system of their host. Poxviruses have evolved multiple, specialised genes to block the various mechanisms used by our cells to respond to infection or warn nearby cells of the danger. But with such specialisation comes restrictions: unlike efficient viruses like Rabies, which infect a whole slew of warm-blooded mammals, the host range of the smallpox virus was limited to humans (the fact that allowed for its successful eradication).
Just as a master strategist will learn from his enemy, so medical researchers may learn from the evolved specialisation of pathogens like poxviruses. By investigating the manner in which poxviruses evade the host immune system, learned by trial and error over the course of thousands of years in their struggle against our bodies’ immune defences, we will gain unexpected insights into how our immune systems work, which may lead to the development of new drugs. What is more, we might also find viral genes that could be used to rein in hyperactive immune systems that cause autoimmune diseases like arthritis. Viruses are not the only ones who can turn genes into double-agents.
Dr Stephen Graham is a Sir Henry Dale Fellow, funded by the Wellcome Trust and the Royal Society, in the Department of Pathology at the University of Cambridge.
Reference
Vaccinia Virus Protein A49 is an Unexpected Member of the B-cell Lymphoma (Bcl)-2 Protein Family
Every moment of every day, our immune systems are battling to keep us healthy against an onslaught from invading organisms. But some of these invaders have evolved to use our very defences against us, writes Dr Stephen Graham, a Sir Henry Dale Fellow.
The text in this work is licensed under a Creative Commons Licence. If you use this content on your site please link back to this page. For image rights, please see the credits associated with each individual image.